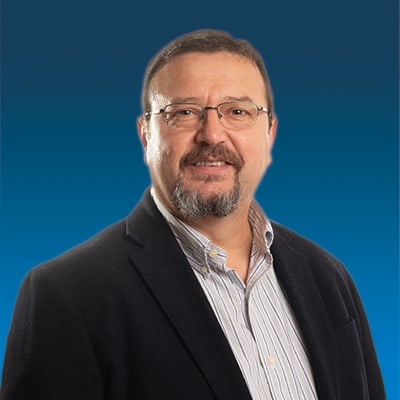
In this series of short technical articles, we look at various topics that illustrate the complexity of renewables as a concept within the ongoing energy transition. Here, PSC’s Zia Emin writes about the complexity that can arise from harmful interactions with increasing network-connected power electronic devices.
Control Interactions – Harmful Oscillations
How networks operate is being changed by factors such as the high penetration of renewable generation, changes in demand, increased use of power electronic devices, use of series capacitors and further HVDC interconnections. Consequently, complexity can arise from harmful interactions with network-connected power electronic devices.
Historically, this area of concern would be categorized as sub-synchronous oscillations (SSO). SSO is a condition where, following a disturbance, the electric power system exchanges a significant amount of energy with a turbine generator at one (or more) of the natural frequencies of the combined system that are below the synchronous frequency of the system[1].
The failure of two shafts at Mohave Generating Station in Southern Nevada in 1970 – caused by the use of series capacitor compensation in AC transmission lines – sparked major research and development on the subject. Three specific interactions between a generator and the electric power system have since been widely investigated:
- Induction Generator Effect (IGE)
- Torsional Interaction (TI)
- Torque Amplification or Transient Torque Effect
Device dependent oscillation
Device-dependent SSO cases can also be observed. These devices range from power system stabilizers (PSS) to HVDC converter controls, static var compensators (SVC), high-speed governor controls and variable speed drives. These are mainly fast power regulation devices. The oscillations that can occur are referred to as Sub-Synchronous Torsional Interactions (SSTI). They are also subject to Sub-Synchronous Control Interaction or Instability (SSCI). While often confused with SSR [2], SSCI doesn’t involve any mechanical interaction and has no fixed frequency of concern.
Another risk factor is the possibility of unintended power electronic system controller behavior and controller interaction. These could be between two HVDC converters, FACTS devices or between an HVDC converter and a FACTS device. This includes devices such as a Static Var Compensator (SVC), a Static Synchronous Compensator (STATCOM), a Unified Power Flow Controller (UPFC) and other similar devices.
Electromechanical oscillations
In all of these cases, we usually focus on electromechanical oscillations (0-3 Hz), small-signal or control oscillations (2-15 Hz), or harmonic resonance analysis (> 15 Hz). The mechanisms of the interaction can be due to control interaction, such as a small disturbance in one DC link causing a sympathetic response in the other via line current commutated HVDC converter stations. Harmonic instability is another interaction category where the converter controls interact with resonances of the network. A classic example is Germany’s BorWin 1, where offshore wind is transmitted to the onshore system via a 400 MW HVDC two-level converter [3].
Real-life examples
An easy way to visualize SSCI is to picture a type 3 (DFIG) wind turbine generator with a series capacitor where effective rotor resistance plays an important role. This was the case in 2009, when an ERCOT system with a transmission line fault left two wind farms radially connected to the system via series capacitors. Following fault clearance, the voltages observed reached 2 per unit and currents were about 300% of normal levels (Figure 1).
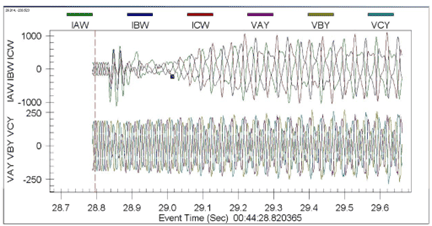
Figure 1 Oscillography recorded at wind system during the event (Image courtesy of Reference [2])
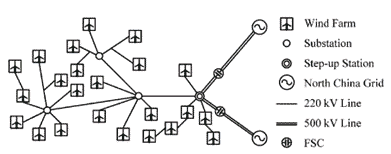
Figure 2 Wind generation and series compensation in Hebei Province (schematic courtesy of Reference [4])
![]() Figure 3 Voltage and current at 220kV of the step-up station (graphs courtesy of Reference [4]) |
![]() Figure 4 Quenching sub-synchronous oscillations by bypassing the series capacitors (graph courtesy of Reference [4]) |
Both the ERCOT and Hebei events saw severe over voltages, current distortion, undamped oscillation, instability, damage to wind farm control circuits, tripping of additional circuits and failure of crowbar circuits (surge arrestors that short-out power supply during an overvoltage condition).
Analyzing the problem
Clearly, there’s a need to minimize the risk of harmful oscillations when planning the system. Several study methodologies have been developed over the years. In general, there are three analysis methods: frequency scanning, eigenvalue analysis (or modal analysis) and time domain analysis. Nyquist stability analysis has also been used as part of an impedance-based analysis of SSO between wind farms and HVDC systems.
How analysis methods compare:
How to mitigate oscillation
Each potential interaction or event has its specific parameters, network topology, triggers and outcomes. So, not surprisingly, no universally accepted mitigation method would satisfy all possible interaction scenarios.
So, in effect, it’s impossible to design control systems that consider all eventual. Advanced analytical methods – such as using real-time digital simulators to test different options and using advanced communication protocols to minimize any external interference – should therefore be part of system planning, asset acquisition and implementation.
For SSCI and SSTI in wind farm generators, solutions can be categorized into one of three categories:
- Supplementary damping controllers (either at GSC or RSC of the turbine)
- Auxiliary damping schemes with FACTS (SVC, STATCOM, etc.)
- Control of series capacitors or isolation of wind generation
The first two methods involve detailed tuning of additional control loops or controllers. These are generally complicated and involve a lot of advanced techniques. The last method simply deals with detection, which leads to switching series capacitors on or off, or the coordinated tripping of a wind farm.
Final words
There is a high potential for interactions between various power electronic-driven equipment or unintended control responses to external system events.
Analytical approaches are still under development, as is work on establishing an accepted structured analysis process.
Mitigation strategies, some easier to implement than others, are available once a possible occurrence is identified. At the moment, the use of a real-time simulator environment – with the ability to model likely scenarios and control strategies – seems to provide an enhanced level of insight in identifying possible interactions or mis-operations.
Overall, it’s important to recognize that – as the energy transition continues – we will encounter technical complexities such as this. But, these challenges should be seen as inevitable bumps in the road rather than roadblocks.
This article is the first in a series of short articles covering various angles related to the complexity of renewables. Check out other topics here.
If you’re interested in being notified when new articles in this series are published, please let us know by clicking here.
References
[1] | IEEE Committee Report, “Terms, Definitions and Symbols for Subsynchronous Oscillations,” IEEE Transactions on Power Apparatus and Systems, Vols. PAS-104, pp. 1326-1334, June 1985. |
[2] | J. P. Lawrence C. Gross, “Sub-Synchronous Grid Conditions: New Event, New Problem, and New Solutions,” in Western Protective Relay Conference, Spokane Washington, October 2010. |
[3] | C. Buchhagen, C. Rauscher, A. Menze and J. Jung, “BorWin1 – First Experiences with harmonic interactions in converter dominated grids,” in International ETG Congress, Bonn, Germany, November 2015. |
[4] | L. Wang, X. Xie, Q. Jiang, H. Liu, Y. Li and H. Liu, “Investigation of SSR in Practical DFIG-Based Wind Farms Connected to a Series-Compensated Power System,” IEEE Transactions on Power Systems, vol. 30, no. 5, pp. 2772-2779, September 2015. |
Series links
The complexity of renewables, Part 1 – PSC Consulting
The complexity of renewables, Part 2 – PSC Consulting
The complexity of renewables, Part 3 – PSC Consulting
The complexity of renewables, Part 4 – PSC Consulting
The complexity of renewables, Part 5 – PSC Consulting
The complexity of renewables, Part 6 – PSC Consulting
The complexity of renewables, Part 7 – PSC Consulting
The complexity of renewables, Part 8 – PSC Consulting
The complexity of renewables, Part 9 – PSC Consulting