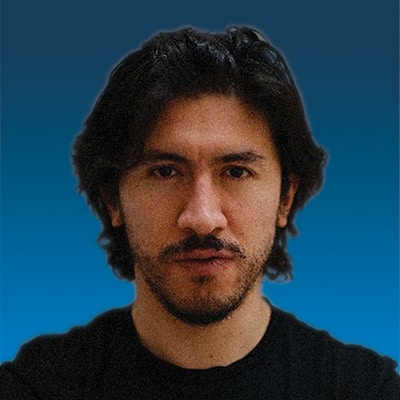
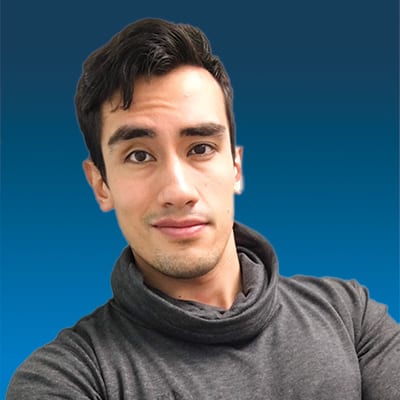
Carlos Ferrandon-Cervantes & Abraham Alvarez-Bustos
Recently there has been an uptick in countries that are transitioning to an overall low-carbon energy system to obtain hydrogen gas from water through a process called water electrolysis. This process involves passing an electrical current through a solution of water and an electrolyte, which causes the water to break down into hydrogen (H2) and oxygen (O2). It is envisaged that the hydrogen produced through electrolysis will be used for various purposes, including fuel cell power generation, transportation, and industrial processes. It can also be stored and transported as a form of energy carrier.
In the UK, a government report, “UK Hydrogen Strategy”[1] describes a range of potential scenarios for the production and use of hydrogen, including different levels of hydrogen production and use in different sectors. The short/mid-term scenario is to reach 42 TWh GW of low-carbon hydrogen by 2030 and 5 GW of low-carbon hydrogen production capacity. This production of hydrogen entails a substantial amount of hydrolysis process in the background. One of the critical research questions is how this “new” load model is incorporated into wider power system models for various studies. Different types of electrolyzers exist[2], namely:
- PEM (Proton Exchange Membrane) electrolyzers.
- Alkaline electrolyzers.
- Solid Oxide electrolyzers (SOE).
- Anion exchange membrane (AEM).
Out of these four types, the PEM electrolyzers have promising power density efficiency and low capital costs, including a smaller footprint and a scalable design.
Classical load modeling
The objective of load modeling is to approximate and imitate the behavior of loads. Load models have been used in power system studies to detail both the steady-state and the dynamic behavior of all loads. Broadly speaking, load models can be classified into two categories, static models, and dynamic models[3]. Static load models express the active and reactive power at any point of the dynamic simulation as functions of bus voltage magnitudes and frequency. There are several types of static load models, including the ZIP model, which represents loads as a combination of constant impedance (Z), constant current (I), and constant power (P) components. The ZIP model is widely used in power system analysis, as it provides a good balance between simplicity and accuracy versus complexity and computational burden.
However, dynamic load models are often necessary to represent certain loads with higher fidelity throughout a dynamic simulation. Furthermore, it is important to understand that depending on the nature of the load, and the penetration level of Inverter-based Renewable (IBR) sources, different variables can be considered if we look at stability concepts, and their behavior is rather different when compared to classical synchronous machine-dominated systems. A high share of IBR sources in a system with low inertia and considerable inductive load can represent challenges for achieving a proper voltage recovery[4]. As such, it is important to consider the behavior of such systems with a considerable amount of electrolyzers.
Load modeling in the context of hydrolysis loads
Classifying the electrolysis load in the power system will depend on the detail and complexity required to model its behavior accurately. In general, the behavior of the electrolysis load can be a combination of static and dynamic components.
At a high level, the electrolysis load can be a static load, as it typically operates at a fixed power consumption level during normal operation. However, the behavior of the electrolysis load can also be influenced by a range of dynamic factors, such as changes in the voltage or frequency of the power supply, changes in the electrolyte concentration, and changes in the temperature of the electrolysis cell. It is worth mentioning that the PEM electrolyzer requires a Power Conversion System with one stage of AC-DC converter and then a DC-DC converter for obtaining the stack current, which is the main control variable of the process. As shown in references[5], the so-called average current mode (ACM) fed into the electrolyzer via the DC-DC converter yields the elimination of the external compensation ramp, an increased gain for current loop at low frequency range, but also a proper sharing of the current among the phases.
These models and dynamic factors can influence the efficiency and stability of the electrolysis process and, depending on the time frame of analysis, can affect their response in a transient stability study.
PSC has the expertise and exciting ongoing projects to further facilitate a transient stability analysis that couples such a relatively new type of load, such as electrolyzers, that can be significant in a future low-carbon scenario.
References
[1] UK Hydrogen Strategy [Online]: https://assets.publishing.service.gov.uk/government/uploads/system/uploads/attachment_data/file/1011283/UK-Hydrogen-Strategy_web.pdf
[2] Tom Smolinka, Emile Tabu Ojong, and Thomas Lickert, “Fundamentals of PEM Water Electrolysis,” in PEM Electrolysis for Hydrogen Production: Principles and Applications, ed. Dmitri Bessarabov, Haijiang Wang, Hui Li, and Nana Zhao (CRC Press, 2015), chap. 6, https://doi.org/10.1201/b19096-6.
[3] Kundur, Prabha S., and Om P. Malik. 2022. Power System Stability and Control. 2nd ed. McGraw Hill.
[4] CIGRE – Stability of inverter-based resource (IBR) dominated systems with different types of local loads [Online] https://cse.cigre.org/cse-n028/stability-of-inverter-based-resource-ibr-dominated-systems-with-different-types-of-local-loads
[5] Tuinema, B.W., Adabi, E., Ayivor, P.K.S., García Suárez, V., Liu, L., Perilla, A., Ahmad, Z., Rueda Torres, J.L., van der Meijden, M.A.M.M. and Palensky, P. (2020), Modelling of large-sized electrolysers for real-time simulation and study of the possibility of frequency support by electrolysers. IET Gener. Transm. Distrib., 14: 1985-1992. https://doi.org/10.1049/iet-gtd.2019.1364